KUIPER BELT
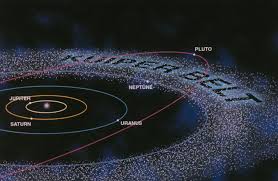
Starting in 1992, astronomers have become aware of a vast population of small bodies orbiting the sun beyond Neptune. There are at least 70,000 "trans-Neptunians" with diameters larger than 100 km in the radial zone extending outwards from the orbit of Neptune (at 30 AU) to 50 AU. Observations show that the trans-Neptunians are mostly confined within a thick band around the ecliptic, leading to the realization that they occupy a ring or belt surrounding the sun. This ring is generally referred to as the Kuiper Belt.
The Kuiper Belt holds significance for the study of the planetary system on at least two levels. First, it is likely that the Kuiper Belt objects are extremely primitive remnants from the early accretional phases of the solar system. The inner, dense parts of the pre-planetary disk condensed into the major planets, probably within a few millions to tens of millions of years. The outer parts were less dense, and accretion progressed slowly. Evidently, a great many small objects were formed. Second, it is widely believed that the Kuiper Belt is the source of the short-period comets. It acts as a reservoir for these bodies in the same way that the Oort Cloud acts as a reservoir for the long-period comets.
The study of the trans-Neptunians is a rapidly evolving field, with major observational and theoretical advances in the last few years. A partial list of relevant papers is included on this Web page. You can also find a Table of the known trans-Neptunians, the discovery images for 1992 QB1, and a blink sequence which shows how these objects are identified.
In 1950, Dutch astronomer Jan Oort proposed that certain comets come from a vast, extremely distant, spherical shell of icy bodies surrounding the solar system. This giant swarm of objects is now named the Oort Cloud, occupying space at a distance between 5,000 and 100,000 astronomical units. (One astronomical unit, or AU, is the mean distance of Earth from the sun: about 150 million km or 93 million miles.) The outer extent of the Oort Cloud is believed to be in the region of space where the sun's gravitational influence is weaker than the influence of nearby stars.
The Oort Cloud probably contains 0.1 to 2 trillion icy bodies in solar orbit. Occasionally, giant molecular clouds, stars passing nearby, or tidal interactions with the Milky Way's disc disturb the orbits of some of these bodies in the outer region of the Oort Cloud, causing the object to fall into the inner solar system as a so-called long-period comet. These comets have very large, eccentric orbits and take thousands of years to circle the sun. In recorded history, they are observed in the inner solar system only once.
In contrast, short-period comets take less than 200 years to orbit the sun and they travel approximately in the plane in which most of the planets orbit. They are presumed to come from a disc-shaped region beyond Neptune called the Kuiper Belt, named for astronomer Gerard Kuiper. (It is sometimes called the Edgeworth-Kuiper Belt, recognizing the independent and earlier discussion by Kenneth Edgeworth.) The objects in the Oort Cloud and in the Kuiper Belt are presumed to be remnants from the formation of the solar system about 4.6 billion years ago.
The Kuiper Belt extends from about 30 to 55 AU and is probably populated with hundreds of thousands of icy bodies larger than 100 km (62 miles) across and an estimated trillion or more comets.
Because KBOs are so distant, their sizes are difficult to measure. The calculated diameter of a KBO depends on assumptions about how reflective the object's surface is. With infrared observations by the Spitzer Space Telescope, most of the largest KBOs have known sizes.
How the Kuiper Belt and Oort Cloud Got Their Names
Both distant regions are named for the astronomers who predicted their existence -- Gerard Kuiper and Jan Oort. Objects discovered in the Kuiper Belt get their names from diverse mythologies. Eris is named for the Greek goddess of discord and strife. Haumea is named for a Hawaiian goddess of fertility and childbirth. Comets from both regions are generally named for the person who discovered them.
Significant Dates
Starting in 1992, astronomers have become aware of a vast population of small bodies orbiting the sun beyond Neptune. There are at least 70,000 "trans-Neptunians" with diameters larger than 100 km in the radial zone extending outwards from the orbit of Neptune (at 30 AU) to 50 AU. Observations show that the trans-Neptunians are mostly confined within a thick band around the ecliptic, leading to the realization that they occupy a ring or belt surrounding the sun. This ring is generally referred to as the Kuiper Belt.
The Kuiper Belt holds significance for the study of the planetary system on at least two levels. First, it is likely that the Kuiper Belt objects are extremely primitive remnants from the early accretional phases of the solar system. The inner, dense parts of the pre-planetary disk condensed into the major planets, probably within a few millions to tens of millions of years. The outer parts were less dense, and accretion progressed slowly. Evidently, a great many small objects were formed. Second, it is widely believed that the Kuiper Belt is the source of the short-period comets. It acts as a reservoir for these bodies in the same way that the Oort Cloud acts as a reservoir for the long-period comets.
The study of the trans-Neptunians is a rapidly evolving field, with major observational and theoretical advances in the last few years. A partial list of relevant papers is included on this Web page. You can also find a Table of the known trans-Neptunians, the discovery images for 1992 QB1, and a blink sequence which shows how these objects are identified.
In 1950, Dutch astronomer Jan Oort proposed that certain comets come from a vast, extremely distant, spherical shell of icy bodies surrounding the solar system. This giant swarm of objects is now named the Oort Cloud, occupying space at a distance between 5,000 and 100,000 astronomical units. (One astronomical unit, or AU, is the mean distance of Earth from the sun: about 150 million km or 93 million miles.) The outer extent of the Oort Cloud is believed to be in the region of space where the sun's gravitational influence is weaker than the influence of nearby stars.
The Oort Cloud probably contains 0.1 to 2 trillion icy bodies in solar orbit. Occasionally, giant molecular clouds, stars passing nearby, or tidal interactions with the Milky Way's disc disturb the orbits of some of these bodies in the outer region of the Oort Cloud, causing the object to fall into the inner solar system as a so-called long-period comet. These comets have very large, eccentric orbits and take thousands of years to circle the sun. In recorded history, they are observed in the inner solar system only once.
In contrast, short-period comets take less than 200 years to orbit the sun and they travel approximately in the plane in which most of the planets orbit. They are presumed to come from a disc-shaped region beyond Neptune called the Kuiper Belt, named for astronomer Gerard Kuiper. (It is sometimes called the Edgeworth-Kuiper Belt, recognizing the independent and earlier discussion by Kenneth Edgeworth.) The objects in the Oort Cloud and in the Kuiper Belt are presumed to be remnants from the formation of the solar system about 4.6 billion years ago.
The Kuiper Belt extends from about 30 to 55 AU and is probably populated with hundreds of thousands of icy bodies larger than 100 km (62 miles) across and an estimated trillion or more comets.
Because KBOs are so distant, their sizes are difficult to measure. The calculated diameter of a KBO depends on assumptions about how reflective the object's surface is. With infrared observations by the Spitzer Space Telescope, most of the largest KBOs have known sizes.
In 1992, astronomers detected a faint speck of light from an object about 42 AU from the sun -- the first time a Kuiper Belt object (or KBO for short) had been sighted. More than 1,300 KBOs have been identified since 1992. (They are sometimes called Edgeworth-Kuiper Belt objects, and they are sometimes called transneptunian objects or TNOs for short.)
One of the most unusual KBOs is Haumea, which is a part of a collisional family orbiting the sun. The parent body, Haumea, apparently collided with another object that was roughly half its size. The impact blasted large icy chunks away and sent Haumea reeling, causing it to spin end-over-end every four hours. It spins so fast that it has pulled itself into the shape of a squashed American football. Haumea and two small moons -- Hi'iaka and Namaka -- make up the family.
In March 2004, a team of astronomers announced the discovery of a planet-like transneptunian object orbiting the sun at an extreme distance, in one of the coldest known regions of our solar system. The object (2003VB12), since named Sedna for an Inuit goddess who lives at the bottom of the frigid Arctic ocean, approaches the sun only briefly during its 10,500-year solar orbit. It never enters the Kuiper Belt, whose outer boundary region lies at about 55 AU -- instead, Sedna travels in a long, elliptical orbit between 76 and nearly 1,000 AU from the sun. Since Sedna's orbit takes it to such an extreme distance, its discoverers have suggested that it is the first observed body belonging to the inner Oort Cloud.
In July 2005, a team of scientists announced the discovery of a KBO that was initially thought to be about 10 percent larger than Pluto. The object, temporarily designated 2003UB313 and later named Eris, orbits the sun about once every 560 years, its distance varying from about 38 to 98 AU. (For comparison, Pluto travels from 29 to 49 AU in its solar orbit.) Eris has a small moon named Dysnomia. More recent measurements show it to be slightly smaller than Pluto.
The discovery of Eris -- orbiting the sun and similar in size to Pluto (which was then designated the ninth planet) -- forced astronomers to consider whether Eris should be classified as the tenth planet. Instead, in 2006, the International Astronomical Union created a new class of objects called dwarf planets, and placed Pluto, Eris and the asteroid Ceres in this category.
While no spacecraft has yet traveled to the Kuiper Belt, NASA's New Horizons spacecraft is scheduled to arrive at Pluto in 2015. The New Horizons mission team hopes to study one or more KBOs after its Pluto mission is complete.
How the Kuiper Belt and Oort Cloud Got Their Names
Both distant regions are named for the astronomers who predicted their existence -- Gerard Kuiper and Jan Oort. Objects discovered in the Kuiper Belt get their names from diverse mythologies. Eris is named for the Greek goddess of discord and strife. Haumea is named for a Hawaiian goddess of fertility and childbirth. Comets from both regions are generally named for the person who discovered them.
Significant Dates
- 1943: Astronomer Kenneth Edgeworth suggests that a reservoir of comets and larger bodies resides beyond the planets.
- 1950: Astronomer Jan Oort theorizes that a vast population of comets may exist in a huge cloud on the distant edges of our solar system.
- 1951: Astronomer Gerard Kuiper predicts the existence of a belt of icy objects just beyond the orbit of Neptune.
- 1992: After five years of searching, astronomers David Jewitt and Jane Luu discover the first KBO, 1992QB1.
- 2002: Scientists using the 48-inch Oschin telescope at Palomar Observatory find Quaoar, the first large KBO hundreds of kilometers in diameter. This object was photographed in 1980, but was not noticed in those images.
- 2004: Astronomers using the 48-inch Oschin telescope announce the discovery of Sedna (2003VB12).
- 2005:Astronomers announce the discovery of 2003UB313. This object, later named Eris, is slightly larger than Pluto.
- 2008: The Kuiper Belt object provisionally known as 2005FY9 ("Easterbunny") is recognized in July as a dwarf planet and named Makemake (pronounced MAHkeh-MAHkeh) after the Polynesian (Rapa Nui) creation god. In September, 2003EL61 ("Santa") was designated a dwarf planet and given the name Haumea after the Hawaiian goddess of fertility and childbirth.
PLEASE GIVE COMMENT.